A Star is Born. What’s Happening on the Front Lines of Fusion, the “Dream” Energy Source
2020/05/13 Toshiba Clip Team
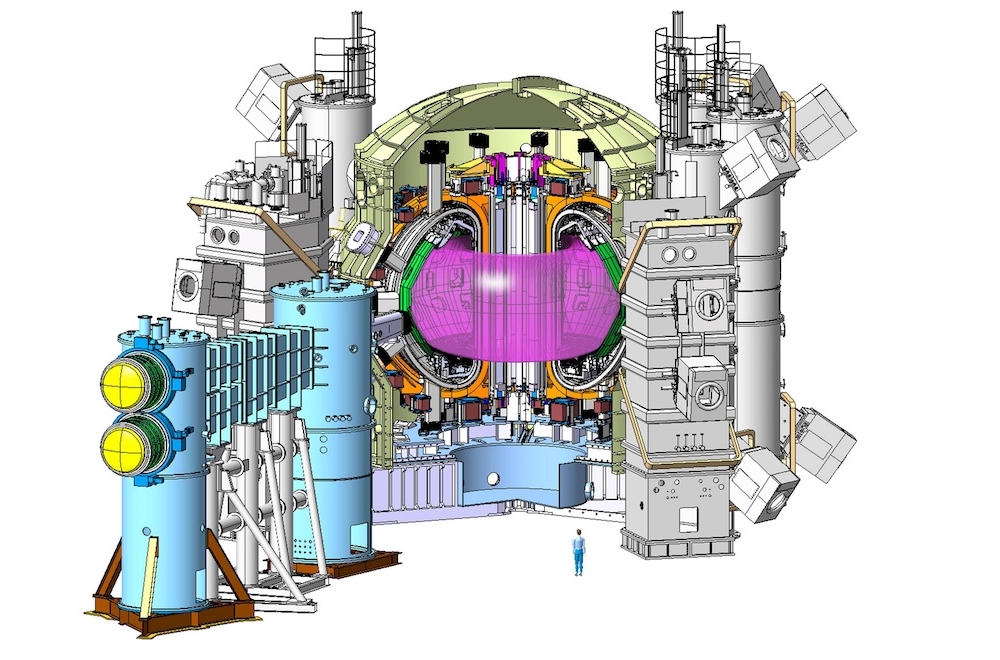
What would it be like to create an artificial “sun” on Earth? What seems like a distant dream of the future, a project too fanciful to comprehend, is in fact already underway. It comes in the form of fusion power—a form of power that is being eyed as the solution to all of our current major energy and environmental problems.
Experimental reactors such as ITER (“The Way” in Latin) and JT-60SA (Super Advanced) are already being built through international cooperation and coordination of various countries, including Japan.
Fusion would be a dream come true, a large-scale, inherently safe, carbon-free source of energy. Here, we head to the front lines of this “dream” energy source to find out the details of these futuristic projects.
Takuma Wakatsuki, Advanced Plasma Experiment Group, Advanced Plasma Lab, Naka Fusion Institute, Fusion Energy Directorate, National Institutes for Quantum and Radiological Science and Technology.
Hideki Kajitani, Superconducting Magnet Development Group, ITER Project Division, Naka Fusion Institute, Fusion Energy Directorate, National Institutes for Quantum and Radiological Science and Technology.
Yusuke Ishii, Advanced System Design & Engineering Department, Toshiba Energy Systems & Solutions Corporation.
A form of power that is highly efficient, highly secure, eco-friendly, with a virtually inexhaustible supply of fuel: How does fusion work?
Fusion, in a nutshell, is about recreating the fusion reactions that occur inside the stars and the sun here on Earth. We first spoke with Takuma Wakatsuki, who researches fusion at the National Institutes for Quantum and Radiological Science and Technology (hereafter “QST”), asking him to explain the process in layman’s terms.
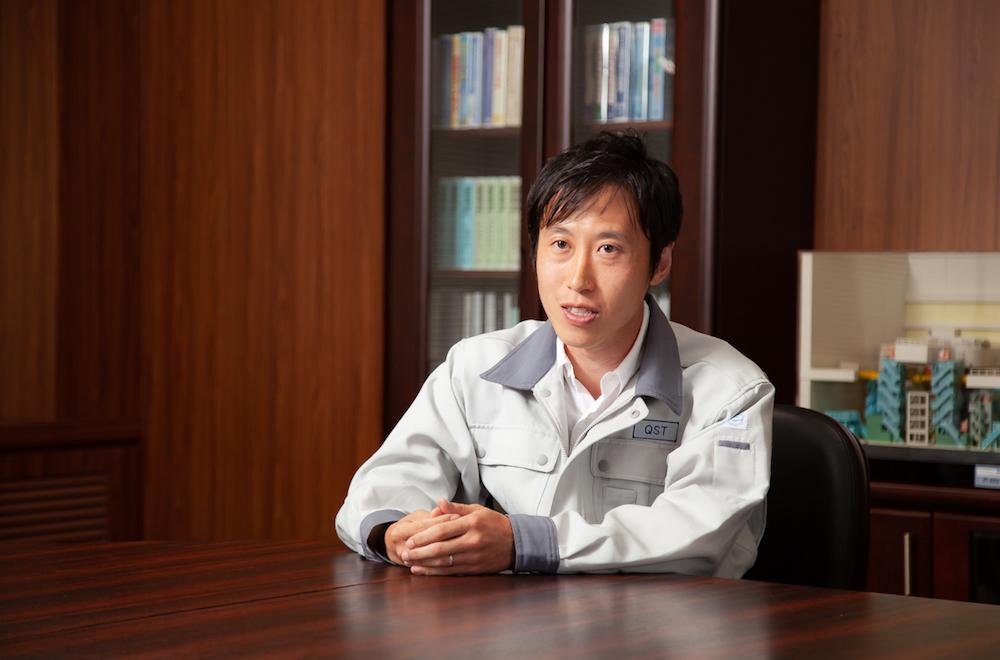
“Everything in the universe is made up of atoms, and these atoms themselves are made up of nuclei and electrons. When these nuclei collide with each other at very high speeds, they fuse into heavier atoms in a process called fusion. You may be unfamiliar with this process, but everyone enjoys the benefits of energy produced in this way. I’m talking, of course, about the source of light that shines down on us every day—the sun. The fusion reactions occurring amongst the hydrogen nuclei in the sun are the source of its heat and light—the same heat and light people enjoy on an everyday basis.
As is evident from the sun, fusion produces a massive amount of thermal energy, which can be used to generate power. The fuel sources of fusion are deuterium and tritium, which are isotopes of hydrogen. With just 1 gram of fuel, fusion can produce the same amount of energy as burning 8 tons of petroleum. There are various methods to generate power, but only (traditional nuclear power plants and) fusion can produce such an enormous amount of energy using a small amount of fuel,” said Wakatsuki.
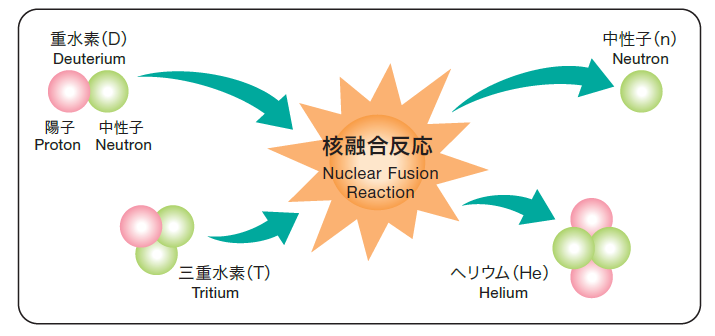
Figure. Mechanism of fusion
The safety of nuclear power has been under question ever since the accident at the Chernobyl Nuclear Power Plant. Fusion, however, is extremely safe.
“Traditional nuclear power plants use thermal energy from fission, which requires several years’ worth of fuel to be stored in the reactors and control rods to control the rate of the nuclear chain reaction. Energy is produced by consuming the fuel little by little over time.
On the other hand, fusion reactors need only the amount of fuel required to sustain the fusion reaction. If the supply of fuel is stopped, the reaction stops. Even if a lot of fuel was brought into the reactor, the fuel itself will drastically cool the plasma*, which will automatically stop the reaction. Fusion, by its very design, does not allow for runaway reactions,” said Wakatsuki.
*Plasma: The fourth physical state of matter, in addition to solid, liquid, and gas. In general, all matter can transform into a plasma state at temperatures of several thousand degrees Celsius or higher (because sufficient energy is provided to free electrons from atoms). Fusion reactors must be capable of generating high-temperature plasmas of more than 100 million degrees Celsius, and contain (maintain) them in a way that does not allow them to touch solids, such as the reactor vessel.
Source: https://www.qst.go.jp/site/qst-english/list39.html
What’s more, fusion does not produce any high-level radioactive waste (fission produces high-level waste) and is a “clean” process, in that it does not emit CO₂.
As if that weren’t enough, deuterium, one of fusion’s fuel sources, can be produced by electrolyzing water, meaning it is virtually inexhaustible. And though fusion’s other fuel source, tritium, is extracted from the environment, it is thought possible to produce it artificially within the reactor, which means in principle, there’s nothing to be concerned about when it comes to the supply chain.
Seven member entities assemble for ITER, the Avengers of the energy world
A form of power that is highly efficient, highly secure, eco-friendly, with a virtually inexhaustible supply of fuel. This, of course, is a dream come true. Then why hasn’t the dream been realized?
Well, fusion reactors are more complex than anything that has ever been built in human history, and their development—currently ongoing—requires the culmination of various cutting-edge technologies. For example, to produce fusion, first you have to turn the hydrogen fuel into a plasma by separating the electrons from the nuclei. This plasma needs to be created in a vacuum and be heated to temperatures of over 100 million degrees Celsius. The plasma must also be contained and controlled inside the reactor by using powerful magnetic fields. Generating these magnetic fields stably requires the use of superconducting coils.
The components used in fusion reactors are massive, yet require dimensional tolerances in the order of millimeters. Fusion reactors are, in a sense, a collective of various cutting-edge technologies.
The fusion process
There is a worldwide project now underway to make fusion a reality, called ITER (“The Way” in Latin)—the Avengers of the energy world, if you will, with seven member entities (Japan, the EU, Russia, the U.S., Korea, China, and India) working together to build an experimental reactor in Saint-Paul-lez-Durance in southern France.
ITER Schematic
Source: Ministry of Education, Culture, Sports, Science and Technology official website
[http://www.mext.go.jp/a_menu/shinkou/iter/021/005.htm]
Japan is in charge of developing and assembling the superconducting coils, which will produce the powerful magnetic fields required to contain the plasma—an essential step in the fusion process. We asked Kajitani of QST and Ishii of Toshiba Energy Systems & Solutions Corporation a little about this vast international project.
“In ITER, each member has a role in terms of procuring the equipment needed to build the actual ITER machine. Currently, we’re developing and manufacturing components in preparation for First Plasma (start of operation) in 2025. Of these components, we are working alongside Toshiba on the production of the superconducting coils, specifically, the toroidal field (TF) coils, which are extremely important components in fusion reactors. The TF coils are enormous structures, 16.5-meters-tall, 9-meters-wide, and weighing 300 tons. And yet the dimensional tolerances required are only a few millimeters. The manufacturing process is multi-faceted, and each step in the process is extremely complex. In the case of the niobium-tin conductors used in the coil, it took over a hundred hours of heating at 650 degrees Celsius to complete,” said Kajitani.
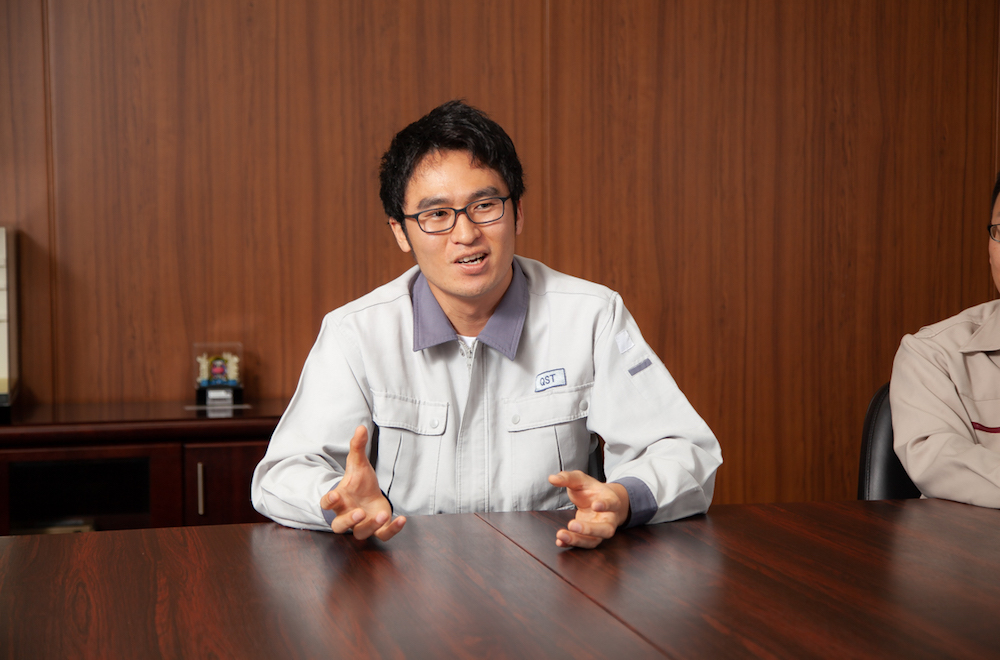
“Toshiba has been involved in the development of fusion technologies since the 1970s. We are involved from the design stage, and have made significant progress in regards to the design and manufacture of these technologies. We have worked with QST to build the fusion device JT-60 (Breakeven Plasma Test Facility), as well as its successor, the JT-60SA (Super Advanced). We’re working to improve the superconducting technologies we have already developed, while also refining the high-precision heat treatment for the conductors in the TF coils for ITER, the measurement process for large-scale structures, and our machining technologies,” said Ishii.
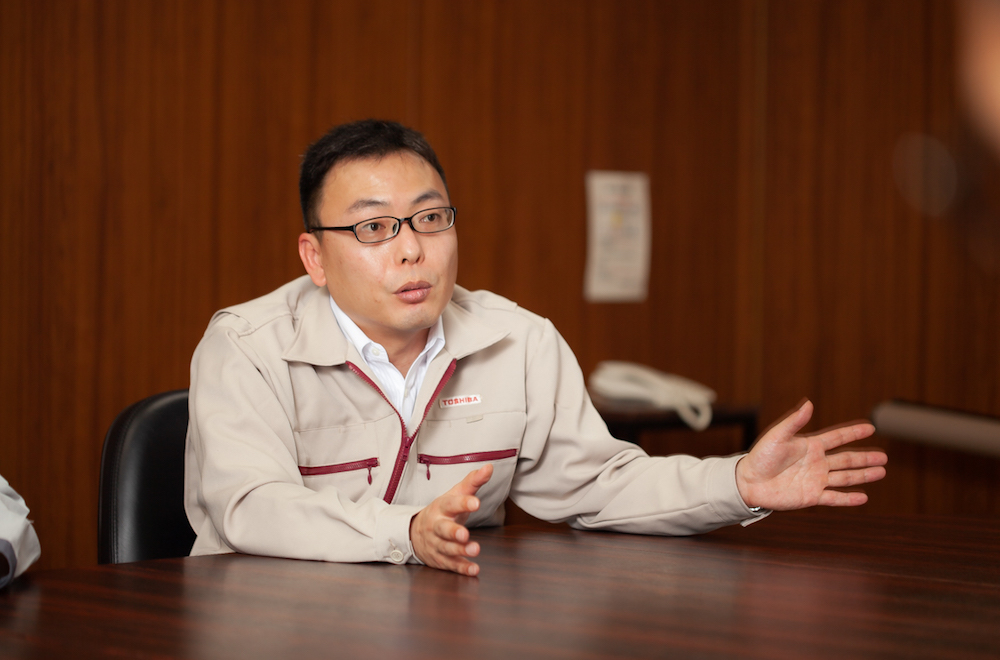
This is a new area of development that combines the field of physics and the engineering sector with manufacturing. The assembly of the TF coils is on a whole other level in terms of the high level of technology required. Though superconducting coils have already been implemented in various kinds of devices, including accelerators, they have never before been implemented at such a large scale with this level of precision.
“To manufacture the TF coils, we need to be knowledgeable and proficient in many various fields. Knowledge of superconductivity, of course, but also electrical engineering and electromagnetics for the coils to achieve the high performance demanded of ITER. Knowledge of fluid dynamics and thermodynamics to analyze the fluid characteristics of the coolant within the coils, and from a manufacturing perspective, material engineering know-how on the various materials involved. The assembly process itself also requires high-level welding and machining technologies. I think these TF coils may be the very first objects of their kind ever created, in terms of involving such high-level technologies from multiple fields,” said Kajitani.
Technology passed down through the generations for the long-fabled dream of fusion
One role of JT-60SA, under joint development of Japan and the EU, is to supplement the research and development for ITER. JT-60SA is an experimental fusion device—the successor of JT-60, which was designed and developed in the 1970s, and JT-60U in the 1980s.
Fusion requires the hydrogen fuel to become a plasma—an ionized state of matter that is similar to a gas. But to achieve fusion, the plasma must be heated to more than 100 million degrees Celsius. JT-60SA will control plasmas by using the magnetic fields generated by superconductors, and under the influence of extreme heat and pressure, will create an ideal plasma environment for fusion research.
“There are three important parameters when it comes to extracting energy from fusion—the temperature, density, and confinement time of the plasma. Right now, we’re trying to figure out how to increase plasma particle density while keeping the plasma confined at higher temperatures for longer periods of time. For ITER, one of our essential missions is to produce burning plasmas using deuterium and tritium. Thus, the design of ITER was based on operation methods that have already been proven to be highly reliable in terms of the three important parameters. However, JT-60SA is designed so that we can conduct more challenging experiments and produce more efficient plasmas. We can increase the pressure of the plasma with respect to the magnetic fields, and conduct more meaningful experiments that will be useful for designing future commercial fusion reactors,” said Wakatsuki.
Experiments for JT-60SA will begin in 2020. If these experiments succeed in stabilizing and maintaining plasmas, it will be a stepping stone towards fusion reactors capable of generating power. There is, in fact, enormous anticipation not just for the international ITER project, but for the cutting-edge research as well.
This high-level research for JT-60SA will also, of course, nudge the assembly of ITER, slated for 2025 operation, steadily forward. And there’s a whole roadmap beyond that—a prototype reactor “DEMO” that can actually produce power through fusion, and commercial reactors that can actually supply electricity to the power grid. Each of our three interviewees, working at the front lines of the development process, emphasized the long-term—how fusion technologies will be passed on to the future.
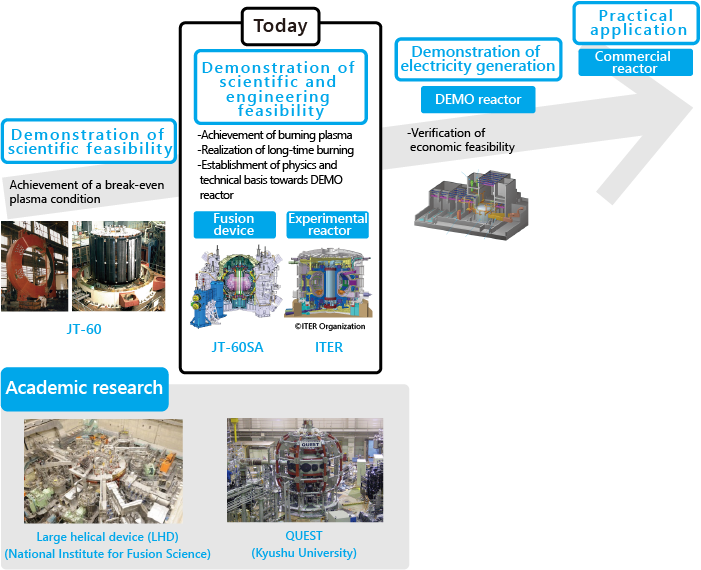
Figure: “Future Fusion Research and Development Strategy” (October of 2005, Advisory Committee on Nuclear Fusion, Atomic Energy Commission) (Courtesy of the National Institutes for Quantum and Radiological Science and Technology/Fusion Energy Research and Development Directorate)
Source: Toshiba Energy Systems & Solutions Corporation
https://www.global.toshiba/ww/products-solutions/nuclearenergy/research/energy.html
“Nowadays, fusion isn’t just some distant dream. It’s a technology that we envision working in the real world. As of now, however, it seems that it’ll only be put into practice—that is, generating electricity—in the mid-21st century, or about 2050. What is essential, in terms of accelerating this research, is skilled personnel. It’s my ever-present hope that we get more new blood—eager individuals that will help drive this world-changing research,” said Wakatsuki.
“There are two types of people involved in developing fusion technologies: people who want to contribute to the realization of a future energy source, and people who want to learn technically demanding technologies and try their hand in a new field. Here at Toshiba, we have many long-time researchers and engineers who have spent their entire lives grappling with fusion technologies. We hope to invigorate their legacy with the zeal of younger generations to turn fusion into a longstanding, deep-rooted project,” said Ishii.
“We’re collaborating with manufacturers to create the TF coils. We’re working as hard as we can towards our goal of delivering all of the TF coils by 2021, but of course, the truly meaningful part of this process lies beyond that. Toshiba has a lot of younger staff working on-site who are very motivated in terms of gaining manufacturing experience, inheriting the torch and building upon cutting-edge technologies. ITER is humanity’s largest-ever international project, and you definitely can’t get this kind of experience anywhere else. It’s an extremely rare thing. I implore the younger staff to make good use of the experiences they gain in ITER in the future. The collective pursuit of developing fusion technologies by us here at QST and Toshiba, and all knowledge gained from it, will surely prove useful in research and development activities beyond the “DEMO” prototype reactor,” said Kajitani.
The many research projects and experiments conducted through ITER and JT-60SA will no doubt result in tier upon tier of new achievements, new hopes, and new dreams. Over the horizon, lying beyond the passion and dedication of these researchers and engineers, is a new energy—something that will perhaps determine the fate of all humanity.
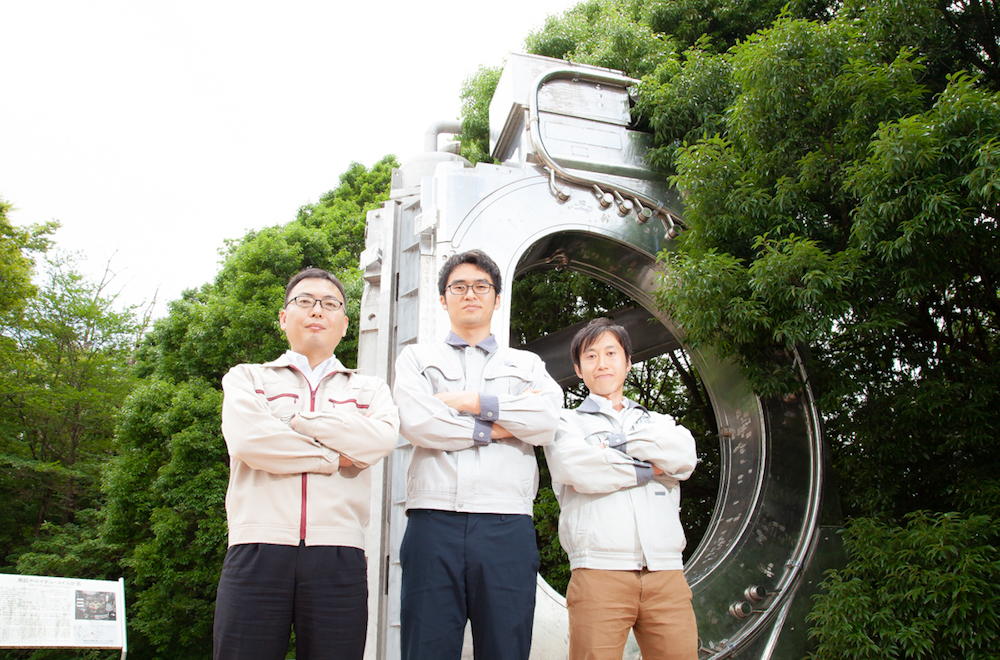