The Climate Crisis Reaches a Watershed: Initiating moves to make CO₂ a resource
2022/08/01 Toshiba Clip Team
- As the climate crisis unfolds, Toshiba is developing technology to reuse CO₂ as a resource.
- Killing two birds with one stone using surplus renewable energy.
- The world’s fastest CO₂ processing speed points the way to commercialization.
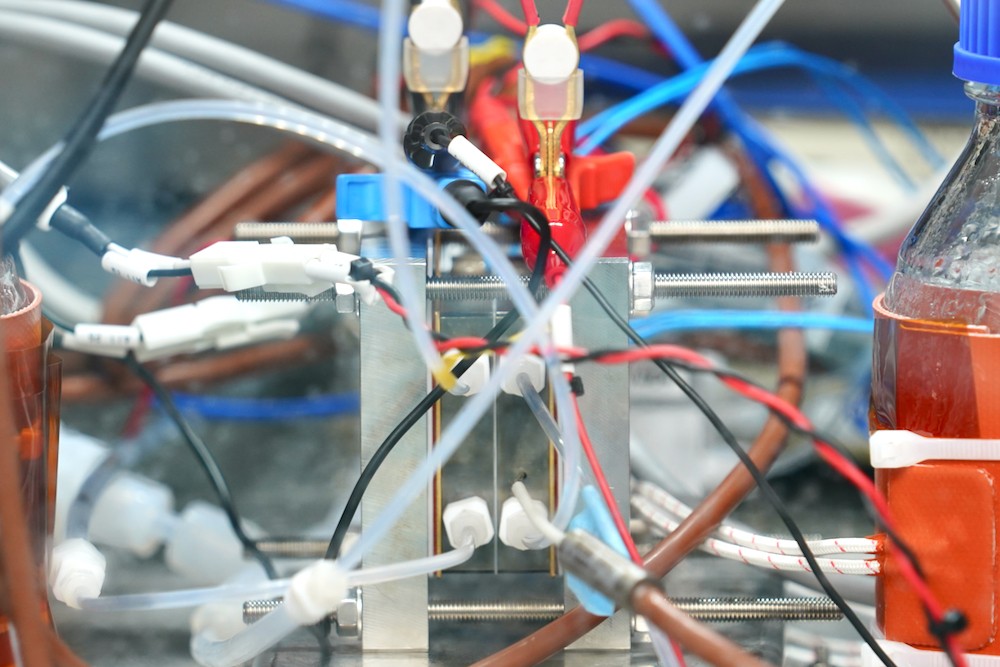
Heavy rain, heat waves, drought—the August 2021 report from Working Group 1 of the United Nations’ Intergovernmental Panel on Climate Change (IPCC) found that, “Human-induced climate change is already affecting many weather and climate extremes in every region across the globe.” On top of this, its “best estimate” was that the average global temperature in the period 2021-2040 would reach a level 1.5°C higher than in 1850-1890, much earlier than previously assumed, and a pointer to future upheavals. The U.S. Department of Energy’s Carbon Dioxide Information Analysis Center estimated that use of fossil fuels and cement manufacturing has released over 400 billion tons of carbon into the atmosphere since 1751. Total CO₂ emissions must be restricted if we are to control global warming.
Toshiba is advancing power to chemicals (P2C) technology that treats CO₂ as a reusable resource. Power from a renewable source is used to decompose CO₂ and carbon monoxide (CO) is collected, for use in the production of synthetic fuel and chemical raw materials. The equipment’s functionality has advanced since the project’s launch in 2011, and now delivers an annual maximum throughput of one ton of CO₂ from an installation area the size of a postal envelope. As of March 2021, that was the world’s fastest processing speed. To find out more about the work, we spoke with the project leader, Koji Mizuguchi, at Toshiba’s Corporate Research & Development Center.
Koji Mizuguchi Senior Manager, Transducer Technology Laboratory, Nano Materials and Frontier Research Laboratories, Corporate Research & Development Center, Toshiba Corporation
Realizing carbon neutrality: using surplus power from renewable energy
“Reducing CO₂ emissions in the industrial sector is essential to achieve carbon neutrality,” says Mizuguchi. “P2C use an electrochemical reaction to convert CO₂ to CO, for use in such areas as chemical production. We identified use of surplus power generated with a renewable energy source as important, so we focused on that early on in our research.”
Surplus renewable energy converts recovered CO₂ to CO for use in chemical products
When the project launched, the IPCC was already warning that global warming would increase with greenhouse gases released by human activities. However, there had been no consensus on an international framework since 1997’s Kyoto Protocol, and there was little interest in CO₂ recovery or recycling. The tide turned with the adoption of the Paris Agreement in 2015. The world made a significant change of direction, toward decarbonization.
“The ‘Green Growth Strategy Through Achieving Carbon Neutrality in 2050’ that Japan’s Ministry of Economy, Trade and Industry announced in December 2020 targets getting 50 to 60% of power from renewable sources by 2050,” Mizuguchi explains. “Until recently, there wasn’t too much concern about surplus power from renewable energy. But renewables fluctuate with natural conditions, like hours of daylight, and their widespread adoption will highlight the need to utilize surplus power to the full. From the very beginning, we planned to utilize this power source.”
Using surplus power from renewable energy in electrolysis coverts CO₂ to CO, a process that kills two birds with one stone, and delivers a raw material for producing chemicals. During the development work, the project team emphasized processing speed—it’s the biggest hurdle on the way to processing large volumes of CO₂ when electrolysis equipment is squeezed into the limited available space in places like power plants and waste incinerators.
The processing speed of the CO₂ electrolysis equipment is key
Advances in high current density, scale and stacking
The team identified three development targets for CO₂ electrolytic cells: high current density, a larger cell area, and stacking to form layers of cells.
Current density measures the charge that flows through a defined areas for a specified time, and the higher the better. Toshiba’s work raised the current from 1.5mA/cm2 to 700mA/cm2, an immense increase of 466 times. A CO₂ catalytic electrode developed by the team played a critical role in this, allowing the team to achieve the world’s fastest CO₂ conversion rate, and opening up the way to equipment miniaturization and lower costs.
The catalytic electrodes are the essence of the P2C process, as it’s the electrochemical reaction that occurs on their surface that drives the CO₂ conversion. Previous approaches have done this with an aqueous solution of CO₂ dissolved in water, but conversion efficiency is low, as the water absorbs miniscule amounts of CO₂. It also requires large, expensive equipment.
The development team came up with a catalytic electrode that uses three phase interface control technology to simultaneously initiate a reaction in the solid (catalyst), gaseous (CO₂) and liquid (water) phases. With this approach, CO₂ in its gaseous state reacts directly with the catalyst, with no need to dissolve it in water, realizing a tremendous increase in CO₂ conversion rates.
Toshiba’s catalytic electrode increases CO₂ conversion rates with smaller equipment at a lower cost
The team’s ingenuity extended to introducing a structure-control technology into the catalyst electrode that gave it a porous structure. This lowers resistance when CO₂ passes through the catalyst and increases of the catalyst area that comes into contact with CO₂.
Three phase interface control technology and structure-control technology that tremendously increase the CO₂ conversion rate
Ryota Kitagawa, development leader for the electrodes, says that the successful outcome was due to “an intuition for finding value even in unexpected discoveries.” It’s a scenario that required the combined forces of fundamental knowledge, flexible thinking, and serendipity.
“Forming the catalyst on the electrode involves a coating process, and we did trials with a range of equipment and various coatings,” says Kitagawa. “I wanted to explore different possibilities, and we ended up selecting equipment that’s not usually used. A surface with regular gaps is generally seen as ideal, but we got an irregular holey structure that would normally be seen as a failure. However, we trusted our instincts as researchers, and when we got the test results we found we had a great conversion rate. We later found that this random structure improved CO₂ flow and gave us an extremely high conversion rate.
Ryota Kitagawa, Expert, Transducer Technology Laboratory, Nano Materials and Frontier Research Laboratories, Corporate Research & Development Center, Toshiba Corporation
While the tenacity not to ignore unexpected discoveries led to a breakthrough that removed one obstacle, Mizuguchi realized that, as he puts it, “The technology was still immature,” and even more CO₂ needed to be processed to achieve practical use. This prompted the team to tune to the other development targets: a larger cell area, and stacking.
The team’s target was to push the size of the electrochemical cell from the current 1 cm2 to between 100 and 400 cm2, and to stack from 10 to 200 cells. The issue here is temperature, as enlarging and stacking cells limits the heat they can radiate away—cell temperatures creep up, hydrogen is generated, and CO₂ conversion falls. Faradaic efficiency provides a measure of how much of an electric charge is available to facilitate electrochemical conversion: without any cooling the cell temperature rose to 50°C and the conversion efficiency was 81%. The team significantly improved on this by investigating the heat generation and optimizing cooling channels between cells. This pulled the cell temperature down to 25 degrees, and Faradaic efficiency went up to 94%.
Cooling channels designed by analyzing heat generation improved CO₂ conversion efficiency.
A future as a resource-exporting country, in sight thanks to independent development
Kitagawa offers his opinion on the development process. “We succeeded in getting a larger area and in stacking cells because we started with the cell structure. Some companies separate material development and device design, but Toshiba has capabilities in many areas, and the skills needed to cover everything from materials to structure. Our team members come from diverse backgrounds, including fuel cells, complex chemistry, and organic chemistry, and that gave us the foundations for carrying out multifaceted simulations, which really drove forward our work.
When the team tested a four-layer cell stack with an area of 100cm2, they recorded CO₂ conversion of 60NL*/h at room temperature, the highest rate in the world. This was realized with compact equipment, only 23cm wide, 13cm deep and 23cm high, which translates to conversion of an annual maximum of 1 ton of CO₂ using equipment with a footprint similar to an envelope. Emissions from a factory that releases 200 tons of CO₂ a day could be handled with an installation area of 2,000 m2, equivalent to five basketball courts.
*Gas volume at standard conditions for temperature and pressure (1 atm. at 0℃)
CO₂ conversion is significantly faster than with artificial photosynthesis cells and conventional cells.
“This result brought CO₂ conversion at the level of a ton a year into sight,” says Mizuguchi. “It’s a touchstone for CO₂ electrolysis, and a passing grade, at least for now. But our target is a processing rate of 100 tons a day. We can do that with cells in arrays of 100 to 1,000. There are still difficulties to get past, but we’ve made strong progress toward practical application.”
The project team is eyeing commercialization of P2C by the late 2020s. With carbon neutrality a fast growing international concern, interest in P2C is starting to gain momentum. In fact, since the verification test results were announced in March 2021, Toshiba has received a constant stream of inquiries.
“With this growing attention in Japan and overseas we have high hopes for collaborations with other companies,” says Mizuguchi. “This is only the team’s idea, but we are wondering if this device can transform Japan, a long-time resource importer, into a resource exporter. That’s our big dream. Our hope is to deliver it to the world, to help solve the climate crisis that humanity faces.
The P2C project team
Note: Departments and titles are as at the time of interviews.
Related Links
*This section contains links to websites operated by companies and organizations other than Toshiba Corporation.
Toshiba’s Carbon Recycling Technology Realizes World’s Highest CO2 Conversion Speed and Achieves Decarbonization in a Limited Space -CO2 electrocatalyst electrode stack technology can convert up to 1.0 ton of CO2 a year at ambient temperatures, with an installation space the size of an envelope- | Corporate Laboratory (Komukai region) | Toshiba