Innovative reactors build on lessons learned from the Fukushima Daiichi accident -Questioning the myth of safety while aiming to coexist with local communities
2024/02/13 Toshiba Clip Team
- Carbon neutrality targets and the international situation prompt reevaluation of nuclear power.
- A mission discovered after repeated self-questioning about working in nuclear energy.
- The iBR: safe for the community; HTGR: combining safety features with multi-purpose use.
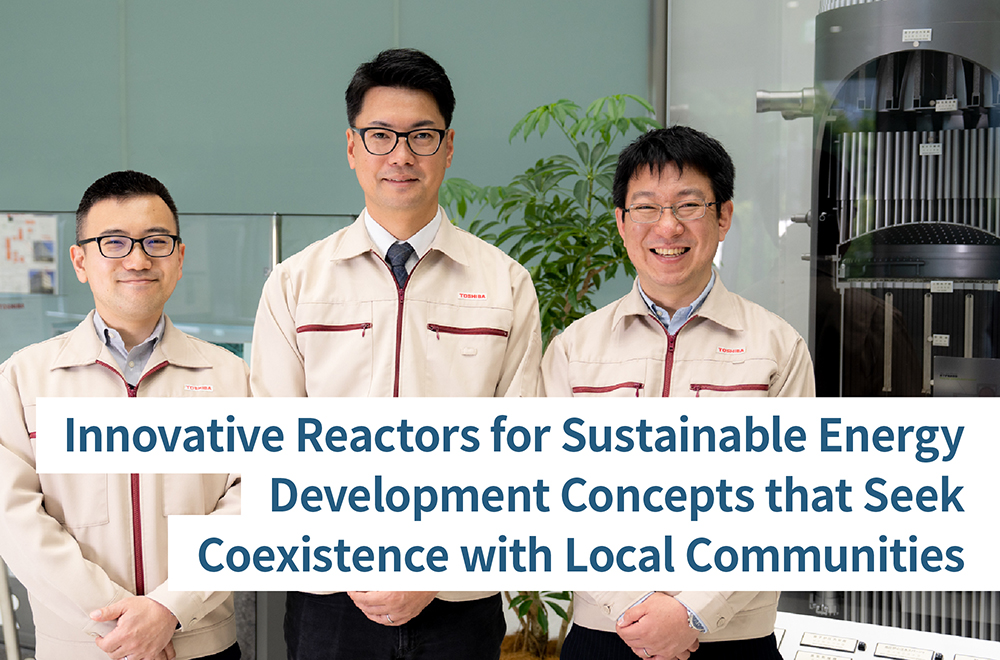
There is a global movement to rethink stable energy supply. One factor in this is Russia’s invasion of Ukraine, but alongside geopolitical risk, the role of energy in addressing carbon neutrality is also a crucial consideration. And that includes nuclear energy.
For instance, the “EU Taxonomy”, which classifies sustainable economic activities, recognizes nuclear power generation as green power, under certain conditions. Japan’s policy on nuclear power is also nearing a major turning point, in the face of the need to maintain a stable energy supply and supply chain. In announcing its Green Transformation (GX) Basic Policy the government said that it would “work on the development and construction of next-generation innovative reactors.”
Toshiba has been working on the development of such innovative reactors since before the March 2011 accident at Tokyo Electric Power Company’s Fukushima Daiichi Nuclear Power Station. Three people on the front lines of the project talk about their mission since the accident: to question the myth of safety and to build on that perspective to create what the Japanese government has designated as “quasi-domestic energy,” which generates power locally with fuel sourced from overseas.
Carbon neutrality and energy uncertainty inspire a review of nuclear power
Yoshio Kawano, who works in external relations for innovative reactors at Toshiba, explains that several countries are starting to rethink nuclear power. “Alongside the global trend toward carbon neutrality, Russia’s invasion of Ukraine has underlined the importance of a stable energy supply, prompting a reassessment of nuclear power. The 2022 G7 summit in Germany reaffirmed the role of nuclear power in the energy mix, and green funds are showing interest, attracted by moves to develop new concept nuclear power plants and the idea of new construction.”
Yoshio Kawano, Specialist, BP Group 2, Business Planning Dept., Power Systems Div., Toshiba Energy Systems & Solutions Corporation
As already noted, Japan is no exception in the movement to reevaluate the importance of nuclear power. The Ministry of Economy, Trade and Industry (METI) announced the Sixth Basic Energy Plan in 2021, which clearly stated that ‘Energy measures are essential for carbon neutrality.’ What this means in practice is that it is necessary to have an energy mix that reviews the composition of energy sources, including solar, nuclear and thermal power. Looking at fiscal year 2030, METI has set the mix at 36-38% renewable energy sources and 20-22% nuclear power.
Repeatedly asking myself if I should continue working in nuclear power brought self-awareness
Nuclear power does not cause accidents. Myths like this about its safety are often repeated. However, the stark reality is that there was an accident at Fukushima Daiichi, which raises questions about how people working on the frontline development of nuclear power plants see the event. For all three men interviewed in this article, the accident at Fukushima happened just after they had joined the company.
Kawano has repeatedly questioned his continued involvement in nuclear power, as have the other two engineers we will meet. However, witnessing the energy supply situation in Japan, and the movement toward realizing carbon neutrality, has led him to the conclusion that “Nuclear power is necessary to support society.” But even as he accepts that he will continue to follow the same course, he is determined to avoid any repetition of the same mistakes.
“There are three major lessons to be learned from what happened at Fukushima Daiichi,” he says. “First of all, strengthen safety measures such as to prevent any occurrence of severe accidents that threaten plant integrity or that could release radiation. Then, second, unlikely though an accident may be, ensure that the plant design can contain the accident and prevent its spread. The third lesson, and perhaps the most important to reflect on for those of us working in nuclear power, including me, is to recognize that no matter how much we strengthen safety measures, we can never reduce the risk to zero.”
Kawano reiterates that he continues to be involved in nuclear power, “Because I believe that nuclear power is indispensable for achieving carbon neutrality and ensuring a more stable domestic energy supply. Our social contributions are the sustenance that make me able to continue my work.”
An innovative reactor with a safety concept that eliminates emergency evacuations and long-term relocation
Even now, more than 12 years later, the accident at the Fukushima Daiichi is still fresh in our collective memory. And we have reached a point where, once again, we are being asked what exactly “safe” means. Of course, the plant was designed with the utmost concern for preventing accidents, but we were forcefully reminded that the power of nature can easily surpass human expectations.
The key consideration is what to do if there is an accident. In an emergency situation like Fukushima Daiichi, it is difficult for even highly trained people to make perfect decisions. This is why innovative reactors are attracting attention.
Kawano offers a definition of what they are: “For Toshiba, innovative reactors need to deliver the high-level safety necessary for coexistence with a local community. In the event that there is a severe accident, they will not trigger emergency evacuations or long-term relocation.”
METI has a classification that covers five categories of innovative reactors: small modular reactors, fast reactors, fusion reactors, innovative light water reactors, and high temperature gas reactors (HTGR). There are different degrees of difficulty to realizing any of them, but each of them is characterized by higher levels of safety and efficiency than current reactors.
“Toshiba has been developing an innovative light water reactor, the iBR, and an HTGR since before the Fukushima accident,” says Kawano. “They meet the needs of our current times, which is why their value has been recognized again. I put it down to our continued development of technologies that improve safety.”
In 2022, Toshiba accelerated development of the iBR and HTGR by setting up the “Innovative Reactor Promotion Team,” a cross-departmental group made up of personnel necessary for innovative reactor development. In parallel with this, a supply chain is being set up to ensure the supply of equipment to Toshiba and to promote technology maintenance.
To find out more about Toshiba’s iBR and HTGR, we spoke to people working on them. First, with Niimi, who is involved in the design of the iBR.
Masayuki Niimi, Specialist, System Planning Group, System Design & Engineering Department, Isogo Nuclear Engineering Center, Power Systems Div., Toshiba Energy Systems & Solutions Corporation
“The iBR’s power generation mechanism is the same as that of boiling-water type light-water reactors,” Niimi explains. “The major difference is that we have greatly improved safety performance. More specifically, the active safety system is stronger, and we have also adopted a passive safety system.”
Schematic of the iBR. Safety innovations that include passive safety systems and a large-capacity, double cylindrical containment vessel to contain hydrogen allow coexistence with communities. It has high electrical output and is economically efficient.
Until now, in the event of a severe accident, boiling water reactors have used active safety systems to cool the reactor core, to prevent it from melting and releasing radioactive materials. This approach relies on pumps and other active equipment. However, the tsunami that hit Fukushima Daiichi took out the emergency power supply, delaying the response. Passive safety systems rely on natural forces such as gravity and pressure differentials for their motive force, and do not need a power source or human intervention. Strengthening the active safety systems of the iBR and adopting passive safety systems has realized problem solving that does not require human intervention or judgment.
“It is a design that embodies lessons learned at Fukushima Daiichi, and reflections on the accident,” says Niimi. “By taking this approach, we aim to reduce the risk of evacuation and land contamination, and to deliver nuclear power generation that can coexist alongside local communities. The iBR passive safety systems can cool the reactor and containment vessel even if emergency power is lost, and the pool that is built into the facility has enough water for seven days of natural cooling. In addition to this, in consideration of natural disasters, the active safety systems have been strengthened by diversifying the power systems, with various distributed emergency power sources in place.
“The worst case scenario is a core meltdown, and to counter this we have introduced innovations that include a double cylindrical containment vessel to confine hydrogen and radioactive materials, and a core catcher that can cool fuel debris as it falls, without any need for power. Even if there is a severe accident, we can confine radioactive materials inside the containment vessel.
With all of these features, the iBR’s safety systems deliver defense in depths with a combination of active and passive safety systems that can ensure safety for seven days without any need for operator intervention—even in the event of a large-scale natural disaster.
The main point is enhanced passive safety that does not require human operation.
The HTGR: Combining excellent safety features with multi-purpose use
While the iBR is an upgraded light water reactor, the HTGR is a new type of reactor that uses fuel coated with silicon carbide or another ceramic, and chemically inert helium gas as coolant. To get more details of the safety features, We spoke to Tetsu Suzuki, who is involved in the design work.
Tetsu Suzuki, Specialist, First Advanced System Design & Engineering Group, Advanced System Design & Engineering Department, Isogo Nuclear Engineering Center, Power Systems Div., Toshiba Energy Systems & Solutions Corporation
“There are three principles that need to be observed to ensure the safe shutdown of a nuclear reactor: Stop; Cool; and Contain.”
“The first of these consecutive steps is to stop all processes in the reactor, to close down the fission reaction. Ordinarily, control rods are inserted into the reactor core to suppress the reaction in the event of an anomaly, such as a large tremor from an earthquake. In the HTGR however, natural physical processes alone automatically suppress the reaction, without any need for control rods.
“The second step is to cool the heat generated by the decay of radioactive materials, which continues even after the reactor has been shut down. The HTGR is cooled by helium gas, but even if, for some reason, the gas does not flow and cool the reactor, the heat from decay is removed passively, by natural heat radiation from the surface of the reactor vessel. Helium is also chemically inert, so there is no risk of the explosions associated with hydrogen and steam. On top of this graphite, which has a large heat absorption capacity, is used for the reactor’s moderator and in other in-vessel structures, realizing the advantage of a slower rise in temperature than with a current light water reactor if there is an accident. All this makes it easier to buy time to cope with any accident.
“The third step, contain, means that even if fuel rods melt, the fuel pellets, fuel rod cladding, pressure vessel, containment vessel, and the outer walls of the reactor building prevent the leaks of radioactive materials. In the HTGR, the fuel particles are coated in a ceramic material that prevents damage to the fuel even at a temperature as high as 1,600°C, and the fuel does not melt because the heat is removed passively.”
Schematic of the HTGR. A natural circulation heat removal system handles heat from radioactive decay. Temperature change is slow, even if there is a severe accident, and no immediate operator action is required.
*Courtesy of Japan Atomic Energy Agency, with some additions and deletions.
Highly safe, because fuel does not melt and there is no risk of hydrogen or steam explosions, even in a severe accident.
Along with safe operation, the HTGR delivers another feature: the supply of heat at a temperature of 750° to 950°C. This high temperature heat can be used for a range of secondary purposes, including seawater desalination, supplying steam to chemical plants, and hydrogen production.
There is already a lot of interest in hydrogen production as a way toward carbon neutrality. There are various ways to produce it, but that produced by a method that does not release CO2 emissions, such as with high temperature heat, is “clean hydrogen,” and it is widely seen as a next-generation energy source. The high-temperature gas-cooled reactor can supply high temperature heat for clean hydrogen production.
“Toshiba is pushing forward with the development of hydrogen-related technologies, and is a recognized leader in high-temperature steam electrolysis. This is a major advantage,” said Suzuki.
Transferring technical capabilities to the next generation by leaving traces
Work on the iBR is advancing, and currently in the process of basic design for implementation. Beyond that lies detailed design and verification testing, and Toshiba’s current plans call for construction and the start operation in the first half of the 2030s.
In Japan, the government has said that it aims to finalize the detailed design of the HTGR by 2030, and to issue permits and construction licenses in the first half of the 2030s. “We expect to start demonstration operations in the second half of the 2030s, with a view to commercialization after that,” said Suzuki. This is the process of technical development needed to achieve carbon neutrality by 2050.
There is a long way to go before we see the commercialization of the iBR and HTGR. Even so, Kawano, Niimi, and Suzuki have resolutely set their sights on the future.
Kawano shows a strong determination: “Innovative reactors will provide a stable energy supply and contribute to carbon neutrality. I want and continue contributing to the project to achieve this goal, and to be involved in more purposeful development work and negotiations.” His two colleagues working to develop the technologies share his sentiment, and we close with their words.
“Development of the iBR has utilized technologies that our predecessors at Toshiba worked hard to create,” says Niimi. “Reactors are very complex systems, so I want to contribute to solving social issues by working as part of the team. I also believe that it is our mission to pass on the technology to the engineers who will follow in our footsteps.”
“Many of our seniors were involved in technology development for a long time, but retired without seeing commercialization,” says Suzuki. “However, they developed excellent technologies that have been passed on to us. Energy security and carbon neutrality are constant goals. The HTGR and iBR should realize those goals. The fact is, the development work takes a very long time, and we have no choice but to move forward steadily. They may not be realized by my generation, but I believe that my role to continue the journey, and leave a mark for the people who follow me.”
More about Toshiba’s Innovative Reactor Development
https://www.global.toshiba/ww/products-solutions/nuclearenergy/research/safety-reactor.html